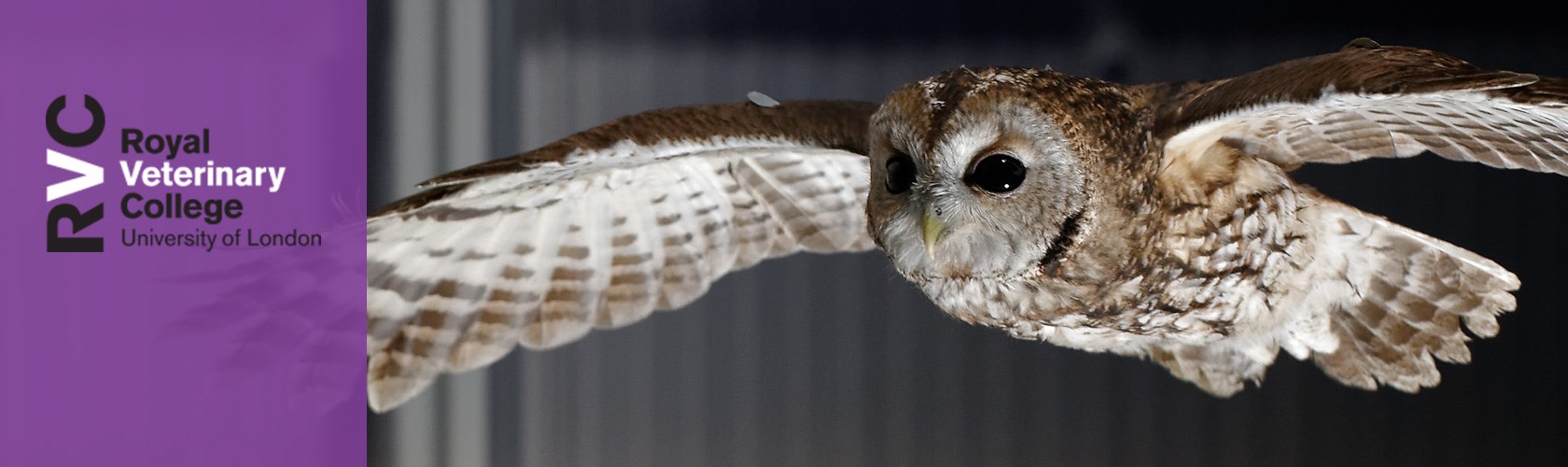
High aerodynamic lift from the tail reduces drag in gliding raptors
Scientists from the RVC and the University of Bristol have discovered how birds are able to fly in gusty conditions – findings that could inform the development of bio-inspired small-scale aircraft.
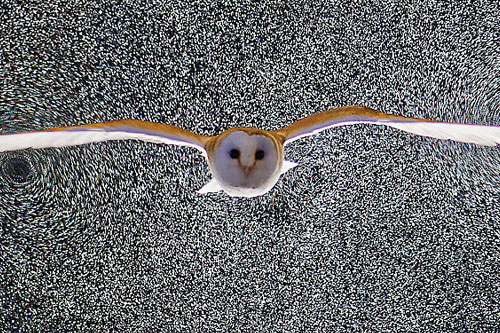
Challenge
Birds and planes must obey the very same laws of physics, and a wing is a pretty good way to create the aerodynamic force known as Lift which balances the Weight of the animal, or aeroplane, due to the relentless pull of gravity. However, there are several notable differences between the two fliers. Flapping is a way to reorient the wings and the aerodynamic force they produce to propel animals forwards in order to balance drag. Drag creates a reduction in speed and planes take a simpler approach to overcoming Drag by using engines for thrust; that may be a jet engine, or a motor-driven propeller. Jets and propellers are relatively rare forms of biological locomotion and not used at all by animals to move through air.
Wings produce the Lift that keeps animals and aeroplanes aloft against the force of Weight. Whether or not a wing is producing Lift it will always experience some Drag from the friction of air as it moves over the wing surfaces. When the wing does start producing Lift, however, Drag begins to rise. Aeroplane and racing car makers constantly search for ways to reduce this Drag while maintaining Lift.
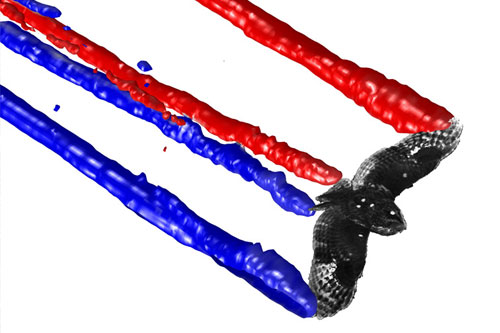
Solution
If birds have evolved the same design for stability as we have discovered for aircraft, then should we see Lift generated by the wings and Downforce generated by the tail? To test this we need is a way to see and measure what the air is doing. Working with LaVision we collaborated to create a method which included filling the lab with soap bubbles containing a little added helium, so that they hung in the air. As the birds glided through this cloud of bubbles, they stirred them up, to measure their speed and direction and understand how the wake moved.
Like racing cars, most passenger aeroplanes also produce Downforce with their tails. In practice, this severely impairs their flight economy because the wings have to produce even more Lift in compensation. Drag increases accordingly, and the engines have to work even harder.
Aircraft designers set tails at that angle for stability. With the wings angled positively (generating Lift) and the tail angled negatively (generating Downforce), in normal ‘trimmed’ flight, the weight of the aircraft must be slightly ahead of the wings. This a setup known as longitudinal dihedral, and allows aeroplanes to recover automatically from disturbances that knock them off course. Should a gust suddenly pitch your aeroplane nose-up, the change in angle means the wings produce a bit more lift and the tail a bit less downforce, leaving a restoring nose-down moment. Equally, if your aeroplane is bumped nose down, the wings produce a bit less lift, the tail a bit more downforce… creating a nose-up restoring moment. This works passively without motors or controllers, and the pilot does not need to take any action to correct the flight path. Instead, the natural mechanics of see-saws and levers sort out the problem automatically.
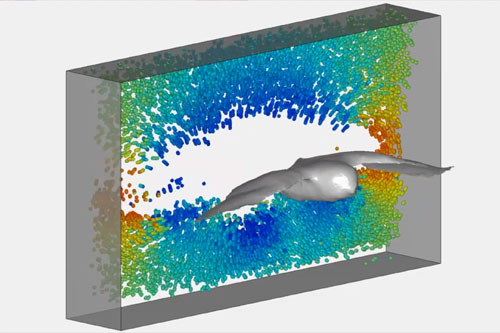
Impact
Birds operate quite differently from aeroplanes. Bird tails generate upward Lift, shown by the bubbles being pushed towards the ground. They forego the aspirational passive stability searched for decades when designing aircraft in favour of a passively unstable configuration. This means that birds must control their pitch angle actively, which takes some processing power, but there are twin advantages to this method. First, passive stability is a trade-off against manoeuvrability, so the birds will be more agile. When they want to pitch, turn or strike at prey they are not fighting against their own stabilisation mechanism. Second, they can greatly reduce their Drag, meaning flight is far more efficient.
Nowadays we have flight controllers that are fast, effective, cheap and lightweight. They appear in every quadcopter drone on the market. Like many others, we believe it is time to use active pitch control in the next generation of aircraft to save energy by increasing efficiency. There are already some large-scale aircraft, such as the Airbus A380 and assorted fighter jets, that generate Lift with their tails, but this trick is increasingly relevant to small-scale aircraft. This is because, for small things moving through the air, the fluid seems relatively more viscous. The component of Drag that birds are minimising is related to the air’s viscosity. This type of drag is comparatively minor for large aircraft because at larger scales the air is relatively less viscous.
Partners
The team at University of Bristol Jonathan Stevenson, Nicholas Durston, Shane Windsor. Lloyd and Rose Buck (www.lloydbuck.co.uk) trained and flew the birds. Resources and support were provided by the Structure and Motion lab at the Royal Veterinary College including Alan Wilson and Maja Lorenc, and by Rosemary Bomphrey and Alice Usherwood.
Publications/Presentations
Title | Publication | Year |
Would planes be better if they were more like birds? Eagle-inspired engineering | The Royal Society Summer of Science | 2021 |
High aerodynamic lift from the tail reduces drag in gliding raptors | Journal of Experimental Biology | 2020 |